Source: https://water.usgs.gov/edu/pesticidesgw.html
Pesticides are chemicals used to kill or control pests such as insects, weeds, fungus, bacteria, rodents, fish or any other type of organism that poses a problem. Pesticides are most often applied to farmland, gardens and lawns. Pesticides are also applied to water bodies (for example, rivers, canals or lakes) to control pests such as mosquitoes, weeds or invasive fish species.
Pesticides have the potential to contaminate drinking water supplies in both agricultural and urban settings. Under the Safe Drinking Water Act (1974), the U.S. EPA and other federal agencies monitor and regulate drinking water supplies. Many contaminants of drinking water occur at very low concentrations. Whether the contaminants pose health risk depends on how toxic the pesticides are, how much is in the water, and how much exposure occurs on a daily basis.
Pesticide contamination of drinking water is very common, especially in agricultural areas. Accidental or illegal spilling or dumping of pesticides can lead to contamination of drinking water, and even proper application of pesticides can lead to contamination of drinking water through leaching into groundwater or runoff into surface water bodies. Concentrations of pesticides tend to be highest in streams adjacent to agricultural areas.
Pesticides can sometimes first appear in drinking water wells decades after the pesticides were applied or spilled, depending on the chemical properties of the pesticide and the geologic conditions.”
In a study published in 2006 by the U.S. Geological Survey, atrazine (a pesticide banned in the European Union but still widely used in the U.S.) was found 90% of the time in streams in agricultural areas and 70% of the time in streams in urban areas, and it was also frequently detected in groundwater [1]. Because groundwater can move very slowly, pesticides can sometimes first appear in drinking water wells decades after the pesticides were applied or spilt, depending on the chemical properties of the pesticide and the geologic conditions. Because of this, even pesticides that are no longer in use can still contaminate water supplies.
PESTICIDE FACTS
- Pesticides are potentially toxic to humans and can have both acute and chronic health effects, depending on the quantity and the ways in which a person is exposed.
- Some of the older, cheaper pesticides can remain in the soil and water for years. They have been banned in developed countries for agricultural use but are still used in many developing countries.
- There are more than 1,000 pesticides used around the world to ensure food is not damaged or destroyed by pests. Each pesticide has different properties and toxicological effects (and the toxicological effects of multiple pesticides can be greater than the sum of their parts).
When using pesticides that may contaminate water supplies, the risk of contamination may be minimized by 1) using short-lived pesticides that biodegrade easily, 2) using pesticides that tend to stick to soil and not move easily, and 3) avoiding disposal of pesticides where they may contaminate water resources, such as near a well or spring or down a storm sewer.
Interesting fact: The pesticide DDT is so persistent in the environment that it is still found in fish more than 40 years after it was banned in the U.S. in 1972.
Health Effects Associated with Pesticides in Drinking Water
There are many different pesticides, each with a different level of toxicity. The health risks associated with pesticides in drinking water are related to how toxic the compound is, how much is in the water, and how much exposure a human gets to the contaminated water. In large doses, which could come from direct exposure to pesticides, they can cause health problems such as cancer, organ damage, reproductive effects, birth defects, or nervous system damage. In drinking water, concentrations are usually low, but some pesticides are toxic even at very low levels.
“High levels of nitrate from chemical fertilizers in the water supply may indicate possible contamination by pesticides.”
Many pesticides are not regulated as contaminants in drinking water, but the U.S. Environmental Protection Agency (USEPA) has set maximum contaminant levels (MCLs) for several pesticides. A MCL is the maximum concentration of a contaminant that is legally allowed in public drinking water systems under the Safe Drinking Water Act. The long-term health risks associated with concentrations above the MCL are considered to be unacceptable. The MCLs for individual pesticides are based on their toxicity, with more toxic pesticides having lower MCLs. Their values range from 0.00005 to 4 milligrams per liter (mg/L or parts per million). For example, the MCL for atrazine, which is the most commonly detected pesticide in drinking water in the U.S., is 0.003 milligrams per litre.
High levels of nitrate from chemical fertilizers in the water supply may indicate possible contamination by pesticides. Because these tests are the expensive and only test for specific compounds, it is best to only test for pesticides that you think may be contaminating your water.
How to Treat Drinking Water for Pesticides
Pesticides can be removed from drinking water by reverse osmosis or granulated activated carbon (GAC) filters. Reverse osmosis works by forcing the water through a membrane that allows water molecules to pass through but blocks larger ions or molecules, such as ones associated with iron, lead or pesticides. In homes, reverse osmosis systems are usually small systems (called point-of-use systems) located near the kitchen sink.
Reverse osmosis systems are cost-effective, but low-end systems can only produce a few gallons of treated water each day. Significant recent improvements in membrane elements allow for more expensive systems to produce 100 or more gallon per day. The taste of the water may be affected by the removal of the minerals.
Granulated activated carbon (GAC) filters are relatively inexpensive and are simple to use. They remove pesticides and other contaminants that stick to small particles of material such as coal or charcoal. These filters can take the form of point-of-use systems or pitchers manually filled with water. GAC filters must be replaced or regenerated periodically to maintain their effectiveness.
FACTORS AFFECTING PESTICIDE POLLUTION OF WATER
Drainage: Farmland is often well-drained and natural drainage is often enhanced by land drains. Water from excessive rainfall and irrigation cannot always be held within the soil structure. Therefore, pesticides and residues (also nitrates and phosphates) can be quickly transported to contaminate groundwater and freshwater supplies over a large geographical area.
The pesticide: Individual pesticides have unique properties, and many variable factors (including those below) determine the specific risk in terms of water pollution.
- active ingredient(s) in the pesticide formulation
- contaminants that exist as impurities in the active ingredient(s)
- additives that are mixed with the active ingredient(s) (wetting agents, diluents or solvents, extenders, adhesives, buffers, preservatives, and emulsifiers)
- degradate that is formed during chemical, microbial, or photochemical degradation of the active ingredient
- Pesticide half-life: The more stable the pesticide, the longer it takes to break down. This can be measured in terms of its half-life, the longer it takes to break down, the higher its persistence. The half-life is unique to individual products but variable depending on specific environmental and application factors.
An active substance is any chemical, plant extract, pheromone, or microorganism (including viruses), that has an action against ‘pests’ or on plants, or parts of plants or plant products.
Mobility in soil: All pesticides have unique mobility properties, both vertically and horizontally through the soil structure. Residual herbicides applied directly to the soil are designed to bond to the soil structure.
Solubility in water: Many pesticides are soluble in the water out of necessity so that they can be applied with water and be absorbed by the target. The higher the solubility of the pesticide, the higher the risk of leaching. Residual herbicides are generally of lower solubility to aid soil binding but their persistence in the soil can cause other problems.
Microbial activity: Pesticides in the soil are primarily broken down by microbial activity. The greater the microbial activity, the faster the degradation. Loss of pesticide residues can also occur by evaporation and photodecomposition.
Soil temperature: Soil microbial activity and pesticide breakdown is largely linked to soil temperature.
Application rate: The more pesticide that is applied, the longer significant concentrations remain.
Application rate: The more pesticide that is applied, the longer significant concentrations remain.
Irrigation Management: Irrigation increases the chance that pesticides will migrate to ground water and surface water. Irrigating saturated soils or irrigating at a rate that exceeds the infiltration rate of soil promotes runoff that can carry pesticides with it. Irrigation that promotes the frequent downward movement of water beyond the root zone of plants also promotes the leaching of substances including pesticides to ground water. This is of particular concern in areas where frequent irrigation is necessary because of coarse-textured soils. Proper irrigation management is critical to minimize the risk of pesticides infiltrating ground water.
How to Prevent Water Contamination
There are a variety of common management practices that provide multiple benefits.
Crop and Soil Management Strategies
- Crop rotation keeps pests off-balance, especially those that prefer a particular crop with its associated cultural practices.
- Cover crops provide crop residues, which enhance soil organic matter.
- Careful crop variety selection ensures that the crop is well-adapted to local conditions and grower needs,and often provides valuable disease or insect resistance, or tolerance to pesticides that will be used to control pests.
- Proper seedbed preparation and planting allows the crop to emerge quickly, potentially reducing early-season disease and insect damage and weed competition.
- Proper drainage and irrigation management promotes optimum plant growth, inhibits various root diseases, and reduces runoff.
- Proper equipment use avoids soil compaction, which can slow crop growth and promote runoff.
Conservation Buffers
Conservation buffers are areas designed to intercept and trap chemicals before they reach surface water. Often native grasses are planted alone or in combination with shrubs and trees along field borders between the crop and a waterway. Buffers trap pesticides, bacteria, fertilizers, and soil sediment, reducing the quantity of potential contaminants that move off the site. Buffers are one of the best management tools a landowner can install, as they offer multiple benefits, and often require little maintenance.
Choose these sites for more information on conservation buffers:
- Conservation Buffers – Design Guidelines for Buffers, Corridors, and Greenways, National Agroforestry Center, USDA
- Conservation buffers can improve water quality, University Extension, Iowa State University
- Use conservation buffers to make dollars and sense, University Extension, Iowa State University
- Buffer Strips: Common Sense Conservation, Natural Resources Conservation Service, USDA
For more on buffers, view or download The Value of Buffers For Pesticide Stewardship and Much More
Integrated Pest Management (IPM)
An IPM program combines the best techniques to prevent pests and to keep them below economically damaging threshold levels and ensure that pesticides are used appropriately. If a pesticide is prone to reach surface or groundwater, suitable IPM tactics can reduce or eliminate the risk of surface or groundwater contamination.
The IPM program also facilitates the selection of a pesticide to be delivered precisely on target and at the proper time. Crop scouting, or monitoring, correctly identifies the pest and collects information needed so that applications are made only when needed, and only when the pest is vulnerable, allowing for a more effective pesticide application. Reducing the need for multiple applications of pesticides reduces the chance that pesticides may reach and contaminate water. Visit the PES site Integrated Pest Management.
Best Management Practices (BMPs) are conservation practices, or systems of practices, and management measures that control soil loss and reduce water quality degradation caused by nutrients, animal wastes, toxics, and sediment. BMPs can improve the environment while also improving the farmer’s bottom line.
Visit any of these web sites to learn more about best management practices to protect water resources from agricultural pesticides:
- Best Management Practices for Agricultural Pesticides to Protect Water Resources, Cooperative Extension, Institute of Agriculture and Natural Resources, University of Nebraska
- Water Publications from Colorado State University,
- http://ohioline.osu.edu/factsheet/fabe-527
- Field Assessment for Water Resource Protection, Purdue University Cooperative Extension Service
- http://www.soil.ncsu.edu/publications/Soilfacts/AG-439-31/
- http://cfpub.epa.gov/npdes/stormwater/menuofbmps/
Selecting Appropriate Pesticides
Protecting water from contamination requires planning and records. Past pest scouting or monitoring records, along with past pesticide application records, help you select the best controls. Selecting the proper pesticide for the crop, the pest, and the site is important. When a site has groundwater near the surface and the soil is permeable, then the leaching potential of the pesticide must be considered during pesticide selection.
Applicators should read the label to find warnings that tell them that the pesticide may leach. Here is an example of language to look for in the Environmental Hazards section of the label: “This product has properties and characteristics associated with chemicals detected in groundwater. The use of this chemical in areas where soils are permeable, particularly where the water table is shallow, may result in groundwater contamination.” There may also be a “Groundwater Advisory” statement on the label. Many new labels have this statement, which is a critical aid in selecting the right pesticide for the job.
Proper Pesticide Mixing and Loading Procedures
More pesticide spills occur while the pesticide is being measured and mixed than during any other part of a pesticide application. Locate the mixing/loading site away from wells, streams and lakes. Maintain a distance of at least 100 feet (check the pesticide label for more specifics) between the mixing and loading site and wellheads, ditches, streams or other water sources.
Measure, mix and load over an impervious surface, such as a concrete pad, which prevents spills from soaking into the ground. Measure the product carefully to avoid spills. Using a closed transfer system to mix and load pesticides also helps reduce the risk of spills. If you are not using a pad, move the mixing and loading steps from place to place to avoid chemical buildup from accidental splashes or spills (see Pesticide Spills).
Be prepared for spills and have a “spill kit” readily available near the mixing loading area. Never leave a tank while it is being filled, and pay constant attention during filling to prevent overfilling and spilling of the pesticide on the ground. Be disciplined and patient.
Applicators should read the label carefully to find warnings regarding mixing/loading pesticides. Here is a statement found in the Environmental Hazards section of many labels: “Most cases of groundwater contamination involving this pesticide have been associated with mixing/loading and disposal sites. Caution should be exercised when handling this product at such sites to prevent contamination of groundwater supplies. Use of closed systems for mixing or transferring this pesticide will reduce the probability of spills. Placement of the mixing/loading equipment on an impervious pad to contain spills will help prevent groundwater contamination.”
Prevent Pesticide Backflow
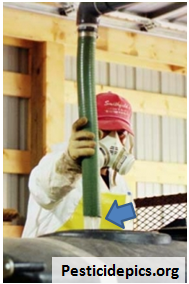
Backflow occurs when a water supply loses pressure and starts flowing backwards toward the water source. The backward flow creates a siphon that draws some of the contents of the sprayer tank back toward the water source if a pipe or hose is below the water surface in the tank. If backflow occurs, the water supply pipes, pumps, and well become contaminated by pesticides from the tank. An anti-siphon device (check valve) prevents backflow and the resulting contamination from occurring. Proper anti-siphoning techniques include the use of a reduced pressure zone (anti-siphon) device or an air gap between the filler pipe and the tank.
Proper Application Procedures
Proper application of pesticides starts with calibration. Calibrating application equipment is the only way to be sure that the proper amount of pesticide is applied. Application of excess pesticide increases the risk of contaminating water by overloading the protective mechanisms of degradation and adsorption, making them ineffective. Over application is not only risky for the environment but is a violation of label directions and the law.
Knowledge of the application site is very important for preventing water contamination. You should know where wells are located, the depth to groundwater, and where surface water is located before making an application. After identifying these features, make plans to protect them. Decide in advance where to turn the application equipment on and off. Using buffer zones and setback areas creates safety zones by keeping applications away from sensitive areas, particularly surface waters. Pesticide applications should hit the target precisely. Applications that move off-target can contribute to water contamination.
Preventing drift is another important task of the applicator. Drifting pesticide can contaminate water and cause other problems. Monitoring the weather conditions, setting the boom height as close as possible to the target, and selecting the proper nozzle type are important activities that help reduce the chance of pesticide drift contaminating surface waters.
Irrigation Management
Irrigation increases the chance that pesticides will
migrate to groundwater and surface water. Irrigating saturated soils or irrigating at a rate that exceeds the infiltration rate of soil promotes runoff that can carry pesticides with it. Irrigation that promotes the frequent downward movement of water beyond the root zone of plants also promotes the leaching of substances, including pesticides, to groundwater. This is of particular concern in areas where frequent irrigation is necessary because of coarse-textured soils. Proper irrigation management is critical to minimize the risk of pesticides moving to groundwater.
Proper Pesticide Storage
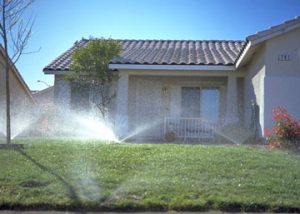
Proper storage of pesticides is also important to prevent water contamination. Locking pesticides inside a fire-resistant, spill-proof facility is an excellent way to prevent accidental pesticide spills. Proper storage is very cheap compared with the expensive consequences of accidents, spills, or fires. Be prepared for spills, and have a “spill kit” readily available inside or near the storage area.
Proper Disposal of Pesticides and Containers
Pesticide containers that have not been triple rinsed pose a risk to water resources. Contaminated containers left outside, and exposed to rain, can leak pesticides into the environment. Triple rinsing pesticide containers prior to disposal remove pesticide residues. Water collected from cleaning and rinsing application equipment should be applied to the original site of the application. Be careful not to exceed label rates. Re-using this pesticide-containing water is an environmentally responsible way to dispose of this material. Collect rinsed containers in a dry, secure, and protected area for disposal. Dispose of the rinsed containers following label directions and local ordinances. Use pesticide container recycling programs where available.
Compiled by Ron Gardner
References
https://www2.usgs.gov/envirohealth/headlines/2015-08-11-understanding_arsenic.html
https://www.usgs.gov/special-topic/water-science-school/science/pesticides-groundwater?qt-science_center_objects=0#qt-science_center_objects
https://www.safewater.org/fact-sheets-1/2017/1/23/pesticides
http://www.filterwater.com/t-pesticides.aspx
https://pesticidestewardship.org/water/prevent-contamination/
https://www.usgs.gov/special-topic/water-science-school/science/pesticides-groundwater?qt-science_center_objects=0#qt-science_center_objects
https://www.safewater.org/fact-sheets-1/2017/1/23/pesticides
http://www.filterwater.com/t-pesticides.aspx
https://pesticidestewardship.org/water/prevent-contamination/