This one is easy to answer; we pretty much agree that you can’t. But
when I think about reasons often given for this barrier I found a flaw
in the reasoning routinely given. The same kind of error was once made
by Einstein himself and later corrected it, the error is called ‘frame
switching’, that is, attributing to one frame the measurement made by
another.
I’m not going to dwell on the incorrect solution but move right along to the correct one.
Let us employ the popular twins of The Twin’s Paradox fame. The twins
initially share the same inertial frame and then one accelerates away.
We know that, from Special Relativity, the mass of the accelerated twin
increases with his speed relative to the stay at home twin. If the
unaccelerated twin is providing the power to push the accelerated twin
then the amount of energy will not increase linearly with increasing
target speed but will rise much quicker as the inertia of the moving
twin increases, eventually rising to infinity.
So we know that we can not accelerate an object to the speed of light that way.
Now we consider the other case, rockets on the accelerated twin’s
vehicle. We note that each twin calculates the same speed difference
between them. Assume that the accelerated twin accelerates in bursts
and then coasts. Each time the accelerated twin coasts he can count
himself stationary and the other twin to be the one that is moving. It
takes no more energy to accelerate by 1,000kmh from any speed that the
twin obtains because at any speed he can count himself stationary and
there is nothing in Special Relativity or physics that can dispute this.
Thus to add another 1,000kph never takes any more energy at any speed,
that is, it does not take any more energy to accelerate from stationary
(at rest with the other twin) or from 280,000kps
What stops the
twin from reaching the speed of light is not some barrier of any kind,
but the problem that he would run out of universe to accelerate in. At
the speed of light the distance between any points in the direction of
travel falls to zero and the interval to travel that distance falls to
zero, so there simply isn’t any more universe left, even an infinite
universe would not solve the problem.
We may try to argue the
addition of velocities but that only applies if an object leaves our
accelerated twin’s rocket at some speed in the direction of travel and
only by the measure of the unaccelerated twin. As far as the
accelerated twin is concerned, he is at rest before each acceleration
step…
What the accelerated twin would notice is that the universe
seems to be getting ever shorter in the direction of his travel, so
there is some indication of motion by that measure. Eventually, the
whole universe would appear to be compacted to a point, so no further
acceleration is possible for that reason.
I have seen written
that as the accelerated twin gains mass it becomes increasingly
difficult for that twin to accelerate. This is not the case. It
becomes more difficult for the unaccelerated twin to push the
accelerated twin (because he measures the increase of mass, the
accelerating twin does not) but not for the accelerating twin that can
accelerate forever, or until he runs out of universe which will occur
before he reaches the speed of light.
The key points are:
1)
The accelerated twin’s inertial frame has the same mass, length, and
temporal frequency (clock rate) regardless of his speed (Einstein said
that all the physics remains the same);
2) At the speed of light the accelerated twin would literally leave the universe for any measurable interval on his clock.
Note also that the energy of the rocket for each acceleration will be
measured differently by the two twins. The unaccelerated twin will
measure each ‘burn’ as taking ever longer as the speed increases but
temperature and pressure decrease (power decreases, thrust decreases),
thus he measures the same energy (power * interval) for each burn.
Note also that if the amount of energy required to accelerate changed
with your speed then, using a fine enough measurement you could
determine when you were absolutely stationary (as this would require the
minimum energy for acceleration) thus establishing a preferred or
absolutely stationary inertial frame, in violation of the most basic
principles of relativity theory ie there is no preferred or absolutely
stationary inertial frame (from which all other frames could be compared
or measured).
To many, the speed of light being the ultimate speed limit is a
fundamental law of physics. Albert Einstein believed that particles
could never travel faster than the speed of light, and doing so would
constitute time travel. For those that regret something in theirpast
this is potentially an interesting question. For those who have led a
completely perfect life, it is an interesting piece of knowledge to have
nonetheless. There are also other reasons to research this question.
The closest star, other than our sun, is Proxima Centauri and is about
twenty five trillion miles away, which would take over ten thousand
years to reach with the fastest spaceship we have today.Therefore if we ever wish to truly explore our universe we must start to explore the limitations of our transport.
To answer this question I must first define a few parameters. As the
question stands it is rather ambiguous. I could say yes, but then slow
light down before conducting my ‘race’, and then show that the particle
did indeed travel faster than the light did. There are many ways in
which this can be achieved, for example a Danish physicist named Lene
Vestergaard Hau was able to slow light down to 17m/s, roughly thirty
eight miles per hour, enabling my car to travel faster than light. They
achieved this by cooling Bose-Einstein condensate atoms to a fraction of
a degree above absolute zero, before passing the beam of light through
it.Obviously
this is cheating, using the the wave-particle duality of light to think
of light as a particle, we know that the light is still travelling at
the speed of light in a vacuum, it just has further to travel, as it
interacts with all the particles in the material. I.e. the light has had
the distance it needs to travel extended, in much the same way as the
resistance of a metal increases when it is heated up, as the electrons
collide more often with the ions in the metal and therefore take longer
to travel through it.Therefore it is only fair to define the speed of
light as the speed of light in a vacuum, known as c, which is 3.00×108
m/s to three significant figures. We commonly slow light down in every
day life, when it bends through glass for example, but does this mean we
can speed it up? Can we make light travel faster than light?
This is known as Superluminal Propagation and is the first
faster-than-light example I would like to talk about. It seems possible
to send pulses of light faster than c over small distances, however
interpreting these results has been difficult because the light pulses
always get distorted in the process.In
2000 Mugnai reported the propagation of microwaves over quite large
distances, tens of centimetres, at speeds 7% faster than c. Impressive
as this is, research by Wang has shown a much larger superluminal effect
for pulses of visible light in which the light is travelling so fast
the pulse exits the medium before it enters it. The observed group
velocity has been calculated as -c/310 . The easiest way to understand
this negative velocity is to interpret this value as meaning the energy
of the wave moved a positive distance over a negative time. In other
words the pulse emerged from the medium before entering it. Although
light can be considered as a particle the photon has no mass, so this is
not a true example of a particle travelling faster than c.
The second thing I will talk about is special relativity andits
consequence, time dilation. The theory of special relativity states that
the speed of light is constant in all reference frames. As a result of
this the speed of light cannot be altered, if I was driving a car at
half the speed of light and I turned on the headlights, the speed of
light would still be measured as c, rather than 1.5c, as might seem
logical. This is perhaps counterintuitive as with much smaller speeds we
would add velocities, for example driving a car at 50mph and throwing a
stone at 20mph in the same direction, would result in the stone having a
velocity of 70mph in that direction. To illustrate time dilation I will
show the effect of moving at high speeds on two clocks.
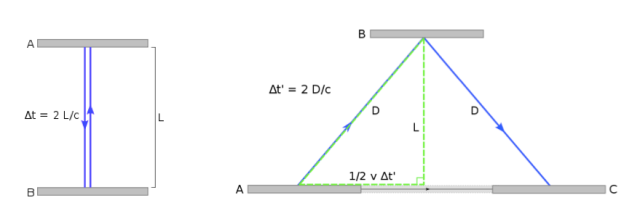
In this situation the clock works by bouncing light between the two
mirrors A and B, this represents one tick, so for the sake of argument I
will say that it takes one second for the light to travel the full
distance between the two mirrors or 2L. Both images depict the same
clock, however the one on the right is moving close to light speed in
relation to the other. The speed of light must be c in both frames,
however as you can see the light has further to travel in the moving
clock. As 2D is longer than 2L, the moving clock appears to be running
more slowly from the frame of the stationary clock. Using these two
examples it is possible to derive the change in time and thus the time
dilation. The first clock gives us the simple equation
and the moving clock shows
. Using Pythagoras theorem we can show that
, this can then be substituted back into the equation and rearranged for
, finally giving .

This expresses the fact that time slows down the faster you go. The
main point of this is that it is possible to travel a distance of one
light year in less than a year. This statement needs explaining however
because for a stationary onlooker, say a person on earth, the journey
time will appear to be much longer than a year. However the traveller
will have experienced less than a year. This is common at CERN where
short-lived particles, such as muons, with a mean lifetime of 2.2
microseconds, are accelerated which hugely extends their lifetime. As we
have no stationary point to measure the universe from, due to the
Earth’s constant movements, we will never have standard time. A clock on
Earth synchronized with one light years away on a distant planet will
not stay in sync for very long, the mass of the planet and its velocity
determine the experience of time. From here I can crudely argue that if
there will never be a standard time the one that matters the most is our
own. Having said that I have not proved that it would be faster than
light, this is a slightly unscientific argument, however it is
interesting to say that if there were no other people in the world to
offer an alternative view I would say that I can travel faster than
light.
My next example involves space-time distortion. After the Big Bang the universe expanded at a rate much faster than 3.00×108
m/s.Special relativity does not provide a limit for distorting
space-time. Miguel Alcubierre hypothesized that a spacecraft could be
enclosed in a ‘bubble’ and exotic matter could be used to rapidly expand
space-time at the back of the bubble, making you move further away from
objects behind you, and contacting it at the front, bringing object
ahead of you closer. This would be a new way of travel in which it would
be space-time which is moving rather than the spaceship. In this way
the ship would reach a destination much faster than a beam of light
travelling outside of the bubble but without anything travelling faster
than c inside the bubble.[6]This
method has one important drawback, It violates the weak, dominant and
strong energy conditions, both the weak and the dominant energy
conditions require the energy density to be positive for all observers,
therefore negative energy is needed, which may or may not exist.[7]
A particle which is always travelling faster than the speed of light
is known as a Tachyon. Tachyons, if they exist, would both answer my
question immediately and have very interesting properties. The equation
has often been used to show that particles with mass can never achieve
the speed of light, this is because it would require infinite energy.
However if the same equation was applied to tachyons, it would show two
things. Firstly, a tachyon would never be able to decelerate below the
speed of light, as crossing this limit from either side would require
infinite energy. Secondly it would have an imaginary mass. When v is
larger than c the denominator in the above equation would become
imaginary, as the total energy must be real the numerator must also be
imaginary. Therefore the rest mass must be imaginary, as an imaginary
number divided by another imaginary number is real.The
existence of tachyons would cause certain causality paradoxes. If they
could be used to send signals faster than c, then if one frame is moving
at 0.6c and another is moving at -0.6c there would always be one frame
in which the signal was received before it was sent. Effectively the
signal would have moved back in time. Special relativity claims the laws
of physics work the same in every frame, if it is possible for signals
to move back in one frame it must be for all of them. Therefore if A
sends a signal to B which moves faster than light in A’s frame and
therefore backwards in time in B’s frame. B could then reply with a
signal faster than light in B’s frame but backwards in time in A’s
frame, thus it could end up that A received the reply before sending the
original message, challenging causality and causing paradoxes.
The mathematical case that prohibits faster than light travel uses the Equation E=MC2,
shows that energy and mass are the same thing, this equation implies
that the more energy you inject into a rocket, the more mass it gains,
and the more mass it gains, the harder it is to accelerate. Boosting it
to the speed of light is impossible because in the process the rocket
would become infinitely massive and would require and infinite amount of
energy.
A wormhole is effectively a shortcut through space-time. A wormhole
connects two places in space-time and allows a particle to travel a
distance faster than a beam of light would on the outside of the
wormhole. The particles inside the wormhole are not going faster than
the speed of light,they are only able to beat light because theyhave a
smaller distance to travel. Scientists imagine that the opening to a
wormhole would look something a bubble. It is theorized that a wormhole
allowing travel in both directions, known as a Lorentzian traversable
wormhole, would require exotic matter. As they connect two points in
both space and time they theoretically allow travel through time as well
as space. This fascinated many scientists and Morris, Thorne and
Yurtsever worked out how to convert a wormhole traversing space into one
traversing time. This process involves accelerating one opening of the wormhole relative
to the other, before bringing it back to the original location. This
uses a process I have mentioned earlier, time dilation. Time dilation
would cause the end of the wormhole that was accelerated to have aged
less. Say there were two clocks, one at each opening of the wormhole,
after this tampering, the clock on the accelerated end of the wormhole
may read 2000 where as the clock at the stationary end showed 2013, so
that a traveller entering one end would find himself/herself in the same
region but 13 years in the past. As fantastic as this may sound there
have been many who believe it would be impossible, there are predictions
that say that a loop of virtual particles would circulate through the
wormhole with ever-increasing intensity, destroying it before it could
be of any use.
Over the course of this essay I have shown a few ways in which the
speed of light can be ‘beaten’, but ultimately I have failed to produce
any proven faster than light particles, and for this reason I must
conclude that particles cannot travel faster than the speed of light.
However the topics I have raised are still in the developing stage, I
have shown that there are many diverse areas of physics which are both
being explored and need exploring. Many of these areas are still heavily
theoretical and are under further research, but our technology is
increasing at an exponential rate and the human race is steadily getting
smarter, so with time and perseverance we will know the answer to
questions such as these and many more. To infinity, and beyond.
Thanks Robert Karl Stonjek